There is one exception at least, one that clearly jumps above all others. This post is dedicated to a bunch of building science specialists - mainly building envelope related - who are brave enough to write and say what they think and do. Their Webpage is called Buildingscience.com. Against all odds, it's not another governmental agency or something paid by a guild of construction materials suppliers. Building Science Corporation is a firm of building consultants and architects, located in Massachusetts with a branch in Ontario. They specialize in building technology consulting, more specifically in preventing and resolving problems related to building design, construction and - yes - operation. They seem to be experts in energy efficiency, buildings retrofit, moisture dynamics, indoor air quality and building failure investigations.
The difference between this team and other building envelope specialists is the people they have and the way they market themselves. Two of the principals, Joseph Lstiburek and John Straube, are also the most active writers of articles in the information part of the Webpage. These guys sum up a huge field experience with strong academic and research roots, combine knowledge with an entertaining writing style, and deal with issues one rarely finds treated with such clarity. Lstiburek founded the company, Straube joined later. Lstiburek seems to be the one with practical roots, reinforced by being part of the 'Building America' program at the US Department of Energy. Straube seems to be the professor in the team, teaching building science in the Civil Engineering Department and School of Architecture at the University of Waterloo, Canada.
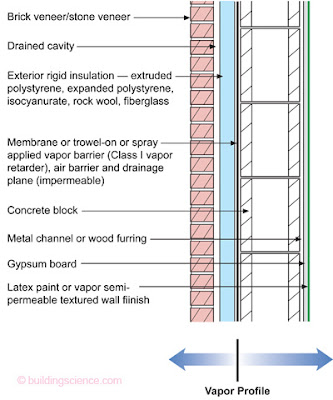
There are several document files available at their Webpage. The most interesting papers can be found under the labels Building Science Digests (BSD), Building Science Insights (BSI), Guides and Manuals (GM) and Research Reports (RR). There is also a complete Glossary of Building Science terms. Digests and Insights are much less dense and really fun to read. Let's have a look for instance at BSI 005: A bridge too far by Joseph Lstiburek. The topic is obviously thermal bridges. You can find sentences as these:
For a bunch of supposedly clever folks we sure do dumb things. One of the big ideas of the past couple of decades or so is to keep the heat out during cooling and keep the heat in during heating. The better we are at this the less energy we need to use to condition the interior. Apparently this concept has not caught on. (...) If an alien from another planet looked at our construction practices he would conclude that we have too much heat in buildings and we want to reject that heat to the outside.
The paper is illustrated with images as clear as the one below (the caption has been copied from the original):
Why can't we be as clear as these folks when discussing about things we all know - and can be measured?
Let's go back to the paper that bears the name of this post, BSI 001: The perfect wall, another example of must read building envelope science. The author is again our entertaining but precise Joseph Lstiburek:
The perfect wall is an environmental separator—it has to keep the outside out and the inside in. (...) Today walls need four principal control layers—especially if we don’t build out of rocks. They are presented in order of importance: a) a rain control layer, b) an air control layer, c) a vapor control layer, and d) a thermal control layer.
In concept the perfect wall (see image to the left) should have the rainwater control layer, the air control layer, the vapor control layer and the thermal control layer on the exterior of the structure. The cladding function is principally to act a an ultra-violet screen and a first rain screen. And yes, architects also consider the aesthetics of the cladding to be important.
Wall to the left is representative of a number of current designs that have been used quite extensively in recent buildings. It is of a basic form consisting of 8-in. back-up and 4-in. facing, in this case stone, which has been widely used in Canada over the past 50 years or more. Insulation is now commonly added to the inside, and may take several forms including mineral wool between strapping or foamed plastic serving also as plaster base. Full mortar backing, which usually requires a very wet mortar, is commonly used behind the stone.
(...) Reference to the winter temperature gradients for Wall to the left will show that all material outside the insulation will fall below freezing. (...) Rain penetration through cracks, occurring as a result of temperature movement in the exterior cladding, can also allow the entry of water and the wetting of the wall.
A dramatic difference in temperature conditions and their attendant dimensional changes can be effected by moving the location of the insulation, see Wall to the right. The main wythe and all the parts of the structure in contact with it are subjected to a much smaller range of temperatures. The possibility of disruptive dimensional changes arising from temperature effects is greatly reduced for all but the exterior cladding and, as will be discussed, these can readily be accommodated. The window frame, now bedded in or fastened to the warm interior wythe, is relieved of the substantial edge-cooling effect of the former arrangement. Advantage can be taken of the inside metal sill to collect and conduct heat to the frame, and a thermal break may be incorporated on the outside to minimize the loss of heat in winter.
![]() |
"Clint Eastwood" Thermodynamics—“The Good” uses offsets and exterior insulation. “The Bad” only uses exterior insulation. “The Ugly” uses neither. |
Why can't we be as clear as these folks when discussing about things we all know - and can be measured?
Let's go back to the paper that bears the name of this post, BSI 001: The perfect wall, another example of must read building envelope science. The author is again our entertaining but precise Joseph Lstiburek:
The perfect wall is an environmental separator—it has to keep the outside out and the inside in. (...) Today walls need four principal control layers—especially if we don’t build out of rocks. They are presented in order of importance: a) a rain control layer, b) an air control layer, c) a vapor control layer, and d) a thermal control layer.
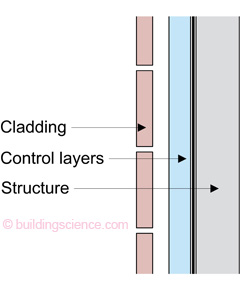
At this point Straube goes for a second to Canada, and refers (without mentioning their names) to the seminal works of the Norwegian O. Birkeland and the Canadian G.K. Garden about the concept of rain screen cladding and the control of rain penetration. I will dedicate a post to these guys and their papers, written in the first half of the 60s, since their influence is greater today than at the time of writing. Another old Canadian professor is cited here, N.B. Hutcheon, whose Principles Applied to an Insulated Masonry Wall (1964) are also completely up to date. The images shown here below, taken from Hutcheon, still resonate in their clarity.
It is interesting to follow Hutcheon's reasoning when he compares these two sections 46 years ago:
(...) The exterior cladding can be arranged as shown for Wall to the right in the form of an open rain screen. It may be set out to form an air space and supported by ledger angles and ties as before. The air space, being heavily vented by suitably designed open joints at both horizontal and vertical intervals, will at all times follow closely the outside air pressure so that the rain screen is substantially relieved of wind pressure differences. This not only removes the major force causing rain to penetrate the cladding, but also eliminates the wind loads on it.
Isn't it amazing? We are still - 46 years later - teaching this exact lesson to new generations of equally astonished architects. Even worse, we still see in 2010 a number of projects with wall sections similar to the left detail instead of to the right one. Lstibureck goes one step further in his paper to discuss the preferred position not just of the thermal barrier, but of the four control barriers as he calls them: rain, air, vapor and thermal. The details are clear in his article. I will summarize here one of the conclussions because it is of great help for us to do a good detail of any building envelope - be it a wall, a roof or a slab in contact with the earth.
Lstiburek describes first the roof adequate build-up (image above to the left) and then the slab in contact with the earth (image to the right), to find out a striking similarity between those two and with a perfect vertical wall:
The perfect roof is sometime referred to as an “inverted roof” since the rainwater control layer is under the insulation and ballast (i.e. roof cladding). Personally I don’t view it as inverted. Those other folks got it wrong by locating the membrane exposed on the top of the insulation—it is they that are inverted. The perfect slab has a stone layer that separates it from the earth that acts as a capillary break and a ground water control layer. This stone layer should be drained and vented to the atmosphere— just as you would drain and vent a wall cladding.
What happens where roofs meet walls?. The classic roof-wall intersection is presented in the figure to the left. Notice that the control layer for rain on the roof is connected to the control layer for rain on the wall, the control layer for air on the roof is connected to the control layer for air on the wall . . . and so it goes. Beautiful. And when it is not so…ugly.
In a beautiful bit of elegance and symmetry if you lie the perfect wall down you get the perfect roof and then when you flip it the other way you get the perfect slab. The physics of walls, roofs and slabs are pretty much the same—no surprise.
This insight was shown into a whole generation of practitioners by the good building envelope specialists since back in the sixties. Where? Our friend Lstiburek is proud to have got it at the University around the eighties - he is a mechanical engineer. Others can not be that lucky: I found this piece of information by myself after finishing my architectural studies. It doesn't matter when - what matters is that, once you get it, you should never again forget it. Articles as clear as this one remind us this lesson. And there are many others at the Webpage... so please, go there and have a look, for your own benefit.